PVD Metal Depostion
E-Beam Evaporation

Filament Evaporation
Sputtering
Alloys
Uniformity and Deposition Rate
Semicircular symmetry allows multiple wafers to be evaporated simultaneously. For liftoff applications, a planar configuration is preferred. A rotating planetary can help with uniformity. Maintaining a lower deposition rate will yield greater uniformity. Placing samples far from the source will help uniformity, but will also lower deposition rate. The deposition rate depends on the position and orientation of the wafer in the chamber. An evaporation rate is the rate at which a material will vaporize (evaporate, change from liquid to vapor) compared to the rate of vaporization of a specific known material. This quantity is a ratio, therefore it is unitless. The equation governing evaporation rate is Revap = , where M = molecular mass, Pe = vapor pressure, k is Boltzman's constant, and T is temperature in Kelvin.

The equation governing deposition rate is Rdep = (see fig. 1). p = density. Note that surfaces parallel with source (theta = 90o) have Rdep = 0. The vapor pressure of a liquid is the pressure exerted by its vapor when the liquid and vapor are in dynamic equilibrium. A substance in an evacuated, closed container, will vaporize a finite amount. The pressure in the space above the substance will increase from zero and eventually stabilize at a constant value, the vapor pressure. Vapor pressures increase with temperature. The boiling point is the temperature at which the vapor pressure of a liquid equals the external pressure. In general, the higher the vapor pressure of a material at a given temperature, the lower the boiling point. In other words, compounds with high vapor pressures form a high concentration of vapor above the liquid. When the vapor source is heated, the vapor pressure of the metal to be evaporated becomes substantial. Hence, atoms are sent out into the vacuum chamber, some of which reach the substrate to form a metal film. Pe =
. s is the surface tension of the molten source, dHv is the enthalpy of vaporization, and N is Avogadro's number. Note that Pe ~ e-T.
A reasonable deposition rate requires a vapor pressure above 10-2 torr. Refractory metals, such as tungsten, can require temperatures in excess of 3000 degC to reach a vapor pressure of 10-2 torr. Other metals, for example nickel, gold and aluminum, require much less heat. Chart of vapor pressures of various metals

Mean Free Path, for purposes of evaporation, is the distance a molecule travels in a straight line (in vacuum) before its velocity vector is randomized by a collision. MFP =, where n = number of moles and d = molecular diameter. This equation represents the average velocity of the molecules times the average time between collisions. Recalling the Ideal Gas law,
, n ~ Pressure (P). Thus MFP ~ 1/P, or as pressure decreases MFP increases.
Step Coverage

Surfaces perpendicular to evaporation source are not coated. Step coverage describes the conformality of a thin film grown over a feature. The aspect ratio (AR) of a step is defined as AR = Step coverage can be improved by
- Planetary with two degrees of rotation.
- Heating the sample substrates. Heating the substrate to ~ 60% of the melting temperature promotes atom mobility after adhesion. This method improves step coverage by making use of surface diffusion. Surface diffusion follows Arrhenius Behavior. With a high enough temperature, the diffusion length can be made larger than the feature size.
In some instances, such as for lift off, it is desirable to achieve zero step coverage. Sputtering will, in general, have superior step coverage (for aspect ratios < .5) as sputtered atoms have random velocities. Evaporated substances tend to be collimated. For high aspect ratios, CVD processes may be used.
Tips for High Deposition Rates
Increase the power.
Quartz Monitor
Quartz crystals are used to monitor deposition rates. The quartz crystal oscillates at a resonance frequency that is dependant on the thickness and mass of the film deposited onto it. When a voltage is applied across the faces of a properly shaped piezoelectric crystal, the crystal is distorted and changes shape in proportion to the applied voltage. At certain discrete frequencies of applied voltage, a condition of very sharp and repeatable electro-mechanical resonance is seen. Quartz monitors are capable of measuring thickness of less than a single atomic layer with 0.5% accuracy. The deposition meter must be programmed for each material it is used to measure. The monitor's position in the evaporation chamber is also relative. The tooling factor is used to calibrate the meter to its position in the evaporation chamber. The quartz crystal must be replaced frequently to provide consistent results. If the deposition rate is oscillating during a controlled deposition, it is a sign that the crystal needs to be replaced. Crystal life is highly dependent on the process conditions: rate, power radiated from the source, location, evaporant, and residual gas composition. To measure deposition thickness precisely, a deposition meter must have knowledge of the material being evaporated, specifically its density and Z-ratio. The Z-ratio is a parameter that corrects the frequency change to a thickness transfer function for the effects of acoustic impedance mismatch between the crystal and the coated material. A table of Z-ratios for common materials is available here. Because the flow of material from a deposition is not uniform it is necessary to account for flux differences between the deposition meter's sensor and any samples. This is accounted for by the tooling factor, which can be experimentally established. Process for determining Density Place a substrate with suitable masking for film thickness measurement adjacent to the sensor, so that the same thickness will accumulate on the crystal and this substrate. Set density of the film material to a tabulated value. Set Z-ration to 1.0 and tooling to 100%. Start with a new crystal and make a short deposition (1000-5000 Ang) and determine the actual thickness. Determine the density with this equation: Density(%) = Dinitial*Tdisplayed/Tmeas. NOTE: tabulated densities are sufficient for most applications. Process for determining Tooling Factor Place a substrate with suitable masking for film thickness measurement adjacent to the sensor, so that the same thickness will accumulate on the crystal and this substrate. Set density and Z-ratio of the film material to tabulated values. Make a short deposition (1000-5000 A) and determine actual thickness. Determine new tooling with this equation: Tooling (%) = TFinitial*Tmeas/Tdisplayed. NOTE: Several evaporations should be made for calibration to average out random variations. Process for determination of Z-ratio Z-values for thin films are in general very close to tabulated bulk values. However, for films under high stress, Z-Values of thin films are slightly smaller than those of bulk materials. Using calibrated density and 100% tooling, make deposition such that half of the crystal lifetime is used. Place new substrate next to sensor and make a second short deposition (1000-5000 A). Determine the actual thickness on the substrate. Adjust the Z-ratio value in the deposition meter to bring the thickness reading in agreement with actual thickness. Troubleshooting with crystals Crystal fails too frequently Crystal is being struck by molten material from evaporation source. Move it further away. Crystal is damaged. Built up material on edge of crystal holder is in contact with the crystal. Large jumps of thickness reading during deposition Crystal is damaged. Crystal is near end of useful life. Crystal holder is dirty. Stress causes film to peel from sensor. Crystal is being struck by molten material from evaporation source. Small pieces of magnetic material are attracted to the sensor. Poor thickness reproducibility Move sensor to different location in chamber. Material does not adhere to the crystal. Sweep, dither, or position where e-beam strikes target has changed. Crystal oscillates in vacuum but not in air Crystal near end of useful life. Excessive moisture accumulation on crystal. Thickness reading changes significantly during source warm up or cool down. Crystal not properly seated. Insufficient cooling.
Vacuum Pumps

Diffusion Pump - high vacuum pumps operating in the range from 10^-3 torr to 10^-7 torr featuring relatively high pumping speed; Diffusion pumps operate by boiling a fluid, often a hydrocarbon oil, and forcing the dense vapor stream through central jets angled downward to give a conical curtain of vapor. Gas molecules from the chamber that randomly enter the curtain are pushed toward the boiler by momentum transfer from the more massive fluid molecules. Removed from high-end applications because of oil vapor backstreaming into the vacuum system and contaminating the chamber. Pressures 10^-5 Torr or lower are referred to as high vacuum (HV). They tolerate operating conditions (e.g. excess particulates or reactive gases) that would destroy other pumps; they have often very high pumping speeds, a relatively low cost, and are vibration and noise free.
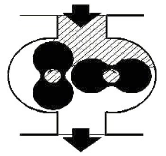
Cryo Pump - an efficient, clean, high-vacuum pump operating in the pressure range from about 10^-3 torr to 10^-10 torr; removes gas molecules from vacuum by trapping them on cold surfaces. Roots Pump - Roots pump blades look like two figure-eights which mesh (without touching) and counter-rotate to continuously transfer gas in one direction through the pump. The blades rely on close mechanical tolerance and high rotational speed (often 3600 rpm) to 'seal' the gases. No oil is used in the direct vacuum path, however it is used to lubricate gears. Occasional oil-vapor leaks through shaft-seals prevents the roots pump from being called strictly oil-free. Roots pumps must be backed by pumps that can exhaust to atmospheric pressure. Roots pumps have a relatively low compression ratio, normally less than 5:1. This ratio varys with the molecular weight of the gases since the atoms/molecules of light gases have much higher average velocities than heavy gases. Light gases can move fast enough to repeatedly collide with the blades and travel the short distance (~ 3 or 4") backwards through the pumping mechanism. More information on the workings of high-vac pumps may be found here.
Summary of Pros and Cons to evaporation methods
Method | Pro | Con |
E-Beam Evaporation | 1. high temperature materials 2. good for liftoff 3. highest purity | 1. some CMOS processes sensitive to radiation 2. alloys difficult 3. poor step coverage |
Filament Evaporation | 1. simple to implement 2. good for liftoff | 1. limited source material (no high temperature) 2. alloys difficult 3. poor step coverage |
Sputter Deposition | 1. better step coverage 2. alloys 3. high temperature materials 4. less radiation damage | 1. possible grainy films 2. porous films 3. plasma damage/contamination |
For details on common materials, liners, melting points, etc. see Thin Film materials reference.
For information on semiconductor substrates, see this link. For thermal properties of pure metals see this link.
Equipment
- Thermal Evaporator
- E-beam Evaporator
- Aluminum
- Chrome
- Nickel
References Kurt J. Lesker Co. for vacuum info. Doolittle, Alan. Physical Vapor Deposition: Evaporation and Sputtering.